UNDERSTANDING MOLECULAR RECOGNITION:
SMALL MOLECULES AS ENZYME INHIBITORS AND TARGETS FOR DNA APTAMERS
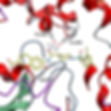
Research Summary
Our main research interests and assignments are focused on identification of novel inhibitors of enzymes involved in lipid metabolism and their evaluation as potential therapeutics. In particular, we are interested in two enzymes, soluble epoxide hydrolase (sEH) and fatty acid amide hydrolase (FAAH). Through traditional medicinal chemistry techniques, including in silico drug design, organic synthesis, structure-activity relationship (SAR) studies and in vitro biological evaluations, our goal is directed toward elucidation of the pharmacology and biochemistry of lipid metabolism and pathophysiology of related diseases.
Development of DNA-based aptamer biosensors
We are using optimized procedure (SELEX) that directly yields DNA-aptameric sensors for small molecules in so-called structure-switching format. We are in particular interested in small molecules such as steroids and drugs that regulate pain and inflammation. Aptamers generated via SELEX hold promise for diverse biomedical applications, such as drug development, bioimaging, drug discovery, disease diagnosis, hazard detection, food inspection, etc.

Inhibitors of sEH and FAAH
sEH ENZYME: Soluble epoxide hydrolase (sEH) is an enzyme that has been detected in various tissues from plants to mammals. In humans it is mostly located in liver, lungs and vascular tissues. This enzyme is selective for aliphatic epoxides of fatty acids, and the best known substrate is epoxyeicosatrienoic acid (EET).
EETs are one of the metabolic derivatives of Arachidonic acid. Under normal circumstances, Arachidonic Acid (AA) is not available for metabolism and is conjugated to the cellular membrane. EETs exhibit vasodilatory effects in various arteries and have also been shown to possess anti-inflammatory properties.
AA is metabolized through three enzymatic pathways, namely, cyclooxygenase (COX), lipoxygenase (LOX), and cytochrome P450 (CYP450) pathways. Whereas the COX and LOX pathways have been studied in detail in the treatment of inflammatory diseases, the third pathway remains unexplored mechanistically and underused clinically.
sEH mediates the addition of water to EETs, leading to the corresponding diols (DHET), which show abolished or diminished or changed biological activity.
Therefore, it has been postulated that sEH inhibition may lead to elevated levels of EETs, which could then have beneficial therapeutic effects on blood pressure, inflammation and other related diseases.
FAAH ENZYME: Endocannabinoids are endogenous lipid ligands that activate the cannabinoid GPCRs CB1 and CB2. These receptors are also activated by THC. It has been known that exogenous CB1 agonists possess properties including pain relief. However, these compounds produce also undesirable side effects including impairments in cognition and motor control, which limit their utility as therapeutic agents. The observation that endocannabinoid synthesis is selectively up-regulated in active neural pathways also suggests that this strategy may have a greater selectivity and lower side effect profile than global activation of cannabinoid receptors via exogenous agonists.
Two endocannabinoids have been identified in mammals: Anandamide (AEA) and 2-arachidonoylglycerol (2-AG). One approach to retain the beneficial effects of cannabinoid activation is to inhibit enzyme that is hydrolyzing endocannabinoids.
FAAH represents an attractive therapeutic target for treatment of pain, inflammation and other CNS disorders.
It is still a major challenge to identify the first inhibitor of FAAH suitable for clinical exploitation that satisfies the requirements of potency, selectivity versus proteins related to anandamide activity as well as other potential off-targets, reversibility versus irreversibility, and efficacy toward rat versus human FAAH.
APTAMERS
Aptamers are synthetic oligonucleotides that can be isolated in vitro against diverse biological targets. Aptamers assume defined 3D structures and generally bind functional sites on their respective targets. Aptamers possess the molecular recognition properties of monoclonal antibodies in terms of their high affinity and specificity. Aptamers are known for their remarkable specificity. Aptamers can discriminate targets on the basis of small differences such as presence or absence of methyl group or the D- vs L-enantiomer.
Because animals or cells are not involved in aptamer identification, toxins as well as molecules that do not elicit a good immune responses can be used to generate high-affinity aptamers. Aptamers are produced by chemical synthesis with extreme accuracy and reproducibility. They are purified under denaturing conditions to a very high degree of purity.
Little or no batch-to-batch variation is expected in aptamer production.
Reporter molecules such as fluorescein and biotin can be attached to aptamers at precise locations identified by the user. Functional groups that allow subsequent derivatization of aptamers with other molecules can also be attached during the chemical synthesis of aptamers.
Aptamers undergo denaturation, but the process is reversible. Once denatured, functional aptamers could be regenerated easily within minutes. They are stable to long-term storage and can be transported at ambient temperature.
Unlike antibodies, aptamers can fold properly and retain activity in the intracellular environment. However, the majority of aptamers with potential therapeutic utility selected to date target extracellular proteins.
The applications of aptamers range from diagnostics and biosensing, target validation, targeted drug delivery, therapeutics, templates for rational drug design to biochemical screening of small molecule leads compounds.
Single-stranded oligonucleotide sequences have an exceptional propensity to assume an array of secondary and tertiary structural motifs with different shapes. This is simply based on the ability of nucleotide bases to interact with each other through Watson-Crick base pairing.

SELEX
Systematic Evolution of Ligands by Exponential Enrichment
The process starts with a chemically synthesized random sequence DNA library containing approximately 10e20 individual molecules (see picture below). A library is designed to contain randomized region (red lines) flanked by two fixed sequence regions (black). Each nucleotide position in the contiguous random region is synthesized upon delivery of a mixture of phosphoramidites containing all four building blocks: A, G, C, and T. To obtain an unbiased library with equal representation of all four nucleotides, the ratios of the four phosphoramidites in the mixture are adjusted based on the coupling efficiencies of individual monomers. Fixed sequences are used for primer binding sites in enzymatic amplification of individual sequences.
A random sequence oligonucleotide (DNA) library is exposed to the target of interest under defined buffer conditions. Sequences that bind to the target are separated from the unbound species by a suitable partitioning technique. This population of sequences recovered from the target (mixture of aptamer candidates) represents a mixture containing both high- and low-affinity binding molecules to the target. Because further screening is required to eliminate the sequences with low affinity binding, individual sequences are amplified for the next round of selection. The aptamer candidate mixture is amplified directly by PCR, provided it is DNA. The single-stranded DNA population obtained by strand separation of PCR products is incubated with a fresh sample of the target for the next round of selection.
The enrichment of the high-affinity sequences at the expense of the low-affinity binders requires several iterations of the selection process carried out under increasingly stringent conditions. Once affinity saturation is observed in an enriched library, the corresponding PCR products are used for cloning and sequencing.
All protocols for selection of aptamers are based on some type of affinity separation of binding and non-binding oligonucleotides, and when it comes to small molecules that often means chemically modifying them and then attaching them to the solid-state matrix. In our protocol, we use small molecules in their native states, that is, unmodified and in solution-phase. It is based on attaching oligonucleotide library via an oligonucleotide complementary to their constant region (PCR primer) to a column, and then passing over the column the target in a solution, thus, isolating oligonucleotides in which the target causes displacement of this complement. After multiple runs of selection, high affinity sequences become dominant – Darwinian selection.

Biosensor
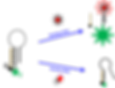
Structure-switching biosensor
When the target (T) is absent, the aptamer binds to quencher-DNA (Q), bringing the fluorophore (F) and the quencher molecule into close proximity for maximum fluorescence quenching. When the target is introduced, the aptamer prefers to form the aptamer-target complex. Upon binding the target, appropriate folding in the random region consequently induces the structural changes, which in turn remove it from the quencher (Q).
DNA origami
DNA origami – a technique for making structures from DNA; nanoscale shapes made from one long single stranded template strand of DNA and hundreds custom short strands (staples) ordered from a DNA-synthesis company (click on the picture below for the full article in Nature, 2006).
Additional Nature Video from 2016 about ten years since the technique was first reported and how DNA origami works and what has been achieved.
DNA Nanorobots
DNA origami, a technique for making structures from DNA, described above, can be also used to build devices, as the researches call them, the nanorobots.
DNA nanorobots can target e.g. cancer cells and deliver an antibody cargo (link to read more). Once this DNA "device" recognizes a cell, it automatically changes its shape and delivers its cargo.
The structure of the DNA nanorobots can be designed using open-source software, called Cadnano. Then the nanorobot can be built using DNA origami technique.
Here is the video of the barrel-shaped DNA nanorobots, each 35 nanometer in size, and having 12 sites on the inside for attaching payload molecules and two positions on the outside for attaching aptamers, short nucleotide strands with special sequences for recognizing molecules on the target cell. The aptamers act as clasps: once both have found their target, they spring open the device to release the payload.
Because the nanorobots can be programmed to release their payload only when the target cell is in the correct disease state, they achieve a specificity that other drug-delivery methods lack, says Hao Yan, a collaborator of Dr. Pecic from Arizona State University, Tempe in this Nature article.